Traumatic spinal cord injury (SCI) leads to persistent deficits below the level of the injury. SCI induces a complex series of spatial-temporal changes in cellular composition, gene expression and extracellular matrix deposition in and surrounding the injury site (Skinnider et al. 2024).
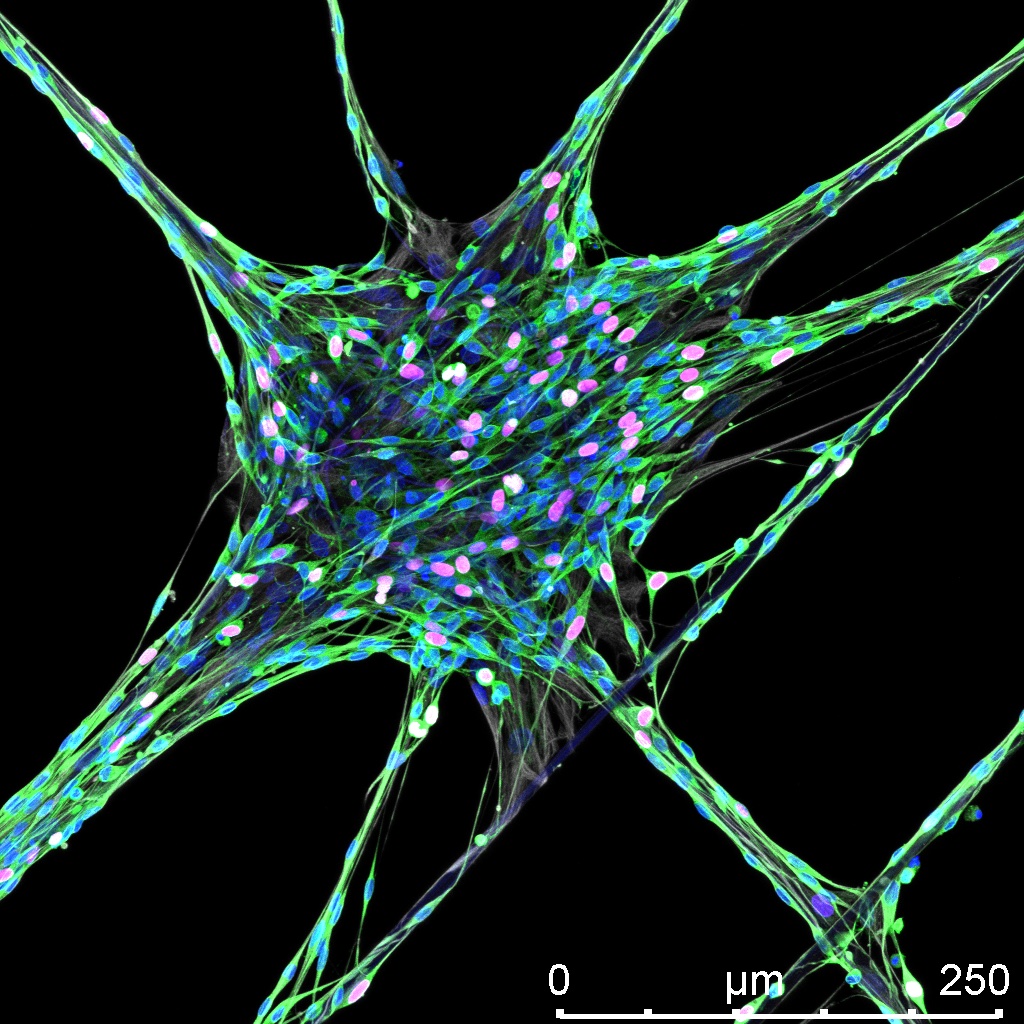
The annual prevalence of SCI in the U.S is greater than 15,000, and patients with persistent paralysis is more than 300,000 (Lee et al., 2014; Injury and Spinal Cord Injury, 2019). Common causes include motor vehicle accidents, falls, sporting accidents and gunshot injuries. Neuronal loss is typically at the site of the injury, but motor symptoms can be extensive below the level of the lesion due to disconnection of descending motor pathways from the brain to surviving motor neurons caudal to the lesion. Development of therapeutic strategies to restore neuronal networks through axonal growth after SCI is an important objective for neurological recovery. In many patients with clinically complete SCI there are bridges of surviving tissue but are not sufficient to sustain significant functional recovery.
In the adult central nervous system (CNS) there is limited axonal growth as compared to the peripheral nervous system (PNS), where under appropriate conditions axons can regrow across anastomosed nerves. This failure of the adult CNS environment to support axonal growth is partially the result of a lack of positive factors such as neurotrophins including BDNF, NT-3 and others (Schnell et al., 1994; Liu et al., 2002). And extracellular matrix components such as laminin. In addition to lack of positive factors, the adult mammalian CNS contains extracellular inhibitors of axonal growth. The primary source of these inhibitors are glial cells, including reactive astrocytes that produce chondroitin sulfate proteoglycans (CSPGs) which impede axonal growth as evidenced by the augmentation of reactive sprouting when these molecules are digested with chondroitinase after SCI (Bradbury et al., 2002; Rosenzweig et al., 2019).
Myelin-forming oligodendrocytes present in the CNS express inhibitory proteins that limit axonal growth in the CNS but not the PNS. These inhibitory myelin proteins include inhibitory Nogo-A, myelin associated glycoprotein (MAG) and oligodendrocyte myelin glycoprotein (OMgp) (Schwab and Strittmatter, 2014). These inhibitor molecules bind to Nogo-66 Receptor 1 (NgR1) (Fournier et al., 2001; Huebner et al., 2011) and inhibit axonal regeneration and sprouting (Schwab and Strittmatter, 2014). In addition to efficacy in rodent SCI models, application of a Nogo receptor decoy molecule promotes limited axonal regeneration and improved functional outcome in SCI in a nonhuman primate model (Wang et al., 2020). This approach is currently in clinical trials with SCI patients (Maynard et al. 2023).
Another approach for SCI therapy is the use of cellular transplantation of cell directly injected into the injury site. Direct transplantation of peripheral myelin forming cells (Schwann cells and olfactory ensheathing cells) has shown some efficacy in preclinical studies (Kocsis et al, 2002; Radtke and Kocsis, 2014). Another cell therapy approach has applied intravenous injection of bone marrow derived mesenchymal/stromal cells (MSCs). Intravenous infusion of MSCs results in functional improvement in SCI rodent models (Matsushita et al., 2015). The treatment results in increased tissue sparing, but also stabilization of the microvasculature with reduced “leakage” in small vessels. A number of potential mechanisms have been attributed to the therapeutic effect of MSCs including production of trophic factor, reduction of inflammation and stimulated axonal growth (Kurahara et al, 2023). Clinical studies in SCI patients are currently ongoing with intravenous infusion of autologous MSCs (Honmou et al., 2021).
It should be emphasized that in the above approaches both preclinical and clinical studies have been carried out in SCI models that were incomplete, i.e. there was some tissue sparing across the spinal cord. The functional improvement in those studies was based on tissue sparing or regenerative processes that could occur via the partially spared tissue at the lesion site. An important but still unachieved approach is to develop an engineered construct that could be implanted into spinal cord injury site that could serve as a matrix to allow axonal regeneration across the lesion site. We have demonstrated in the PNS that spider silk can provide a matrix to allow axonal regeneration. Seeding the spider silk with peripheral myelinating cells can also enhance regeneration.
In the current proposal we will determine if an implanted spider silk-based conduit into the site of spinal cord injury can promote axon growth through and possible out of the construct. If we are able to bridge the gap of the lesion demonstrated by axon growth along the fibers across the lesion this would be a first important step in developing a novel therapeutic effect for SCI. We are aware axons growing into the construct may be impeded by local inhibitory molecules to grow out of the spider silk construct to neurons below the lesion to make synapses for possible improved motor function. This would imply that an additional therapeutic intervention such as co-treatment with Nogo receptor decoy molecules or intravenous
MSC treatment would be required. However, currently with complete transection there no prospect of function improvement. Generally, some tissue sparing is required to see functional improvement after interventional approaches in both preclinical and clinical studies in SCI. Therefore, our primary objective is to determine if could provide a “bridge” using spider silk as a matrix for axonal growth that would potentially mimic tissue sparing.
Research Group
Publications
-
Schnell L, Schneider R, Kolbeck R, Barde YA, Schwab ME. Neurotrophin-3 enhances sprouting of corticospinal tract during development and after adult spinal cord lesion. Nature 1994; 367:170–3.
-
Liu Y, Himes BT, Murray M, Tessler A, Fischer I. Grafts of BDNF producing fibroblasts rescue axotomized rubrospinal neurons and prevent their atrophy. Exp Neurol 2002; 178: 150–64.
-
Bradbury EJ, Moon LD, Popat RJ, King VR, Bennett GS, Patel PN,et al. Chondroitinase ABC promotes functional recovery after spinal cord injury. Nature 2002; 416: 636–40.
-
Rosenzweig ES, Salegio EA, Liang JJ, Weber JL, Weinholtz CA, Brock JH, et al. Chondroitinase improves anatomical and functional outcomes after primate spinal cord injury. Nat Neurosci 2019; 22: 1269–75.
-
Schwab ME, Strittmatter SM. Nogo limits neural plasticity and recovery from injury. Curr Opin Neurobiol 2014; 27: 53–60.
-
Fournier AE, GrandPre T, Strittmatter SM. Identification of a receptor mediating Nogo-66 inhibition of axonal regeneration. Nature 2001;409: 341–6.
-
Huebner EA, Kim BG, Duffy PJ, Brown RH, Strittmatter SM. A multidomain fragment of Nogo-A protein is a potent inhibitor of cortical axon regeneration via Nogo receptor 1. J Biol Chem 2011; 286:18026–36
-
Maynard, George 2023 in Lancet Neurology.You can get online.
-
Radtke and Kocsis 2014
-
Kocsis et al. 2002 (our review on SC and OEC transplantation in VA journal)
-
Honmou O, Yamashita T, Morita T, Oshigiri T, Hirota R, Iyama S, Kato J, Sasaki Y, Ishiai S, Ito YM, Namioka A, Namioka T, Nakazaki M, Kataoka-Sasaki Y, Onodera R, Oka S, Sasaki M, Waxman SG, Kocsis JD. Intravenous infusion of auto serum-expanded autologous mesenchymal stem cells in spinal cord injury patients: 13 case series. Clin Neurol Neurosurg. 2021 Apr;203:106565. doi: 10.1016/j.clineuro.2021.106565. Epub 2021 Feb 18. PMID: 33667953.
-
Kurihara K, Sasaki M, Nagahama H, Obara H, Fukushi R, Hirota R, Yoshimoto M, Teramoto A, Kocsis JD, Yamashita T, Honmou O. Repeated intravenous infusion of mesenchymal stem cells enhances recovery of motor function in a rat model with chronic spinal cord injury. Brain Res. 2023 Oct 15;1817:148484. doi: 10.1016/j.brainres.2023.148484. Epub 2023 Jul 11. PMID: 37442249.